Overview
Materials and Systems Engineering Lab (MaSEL) is passionate about developing designer materials and novel processes to address DOE energy earthshots such as Carbon Negative, Hydrogen, and Long Duration shots, as well as NAE Grand Challenges (GC), including manage the nitrogen cycle, develop carbon sequestration methods, engineer better medicines, and engineer the tools of scientific discoveries. Our overall approach toward the discovery of new materials and process innovation is shown in Fig. 1.
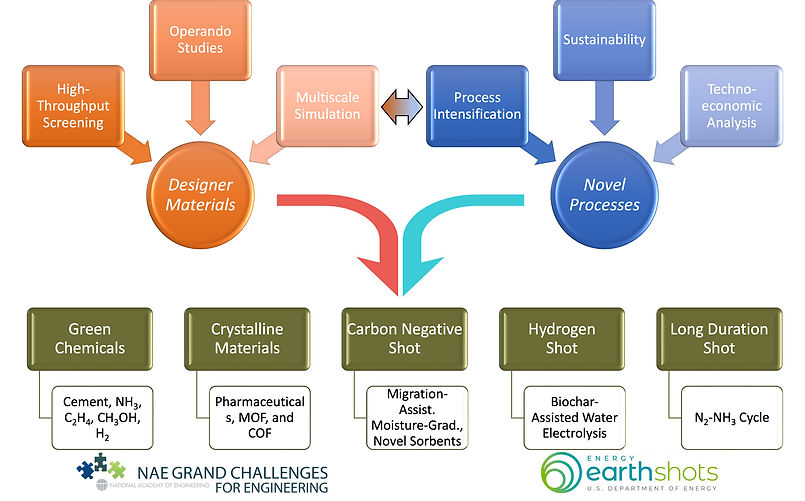
Figure 1: A convergent research scheme combining material discovery cycle (orange color) and process innovation (blue color) to address NAE GCs and DOE Energy earthshots.
Discovery of Materials
Our discovery cycle begins with the initial material targets which are obtained from ab initio computations such as density functional theory. We have a strong collaboration with computational scientists – namely, Dr. Santanu Chaudhuri (Argonne National Lab [ANL], Area: porous materials), Dr. Anh Ngo (ANL, Area: sorbents and catalysts), Dr. Jindal Shah (Oklahoma State University, Area: sorbents), Dr. Jason Goodpaster (University of Minnesota [UMN], Area: catalysts), Dr. Joseph Gauthier (Texas Tech. University [TTU], Area: catalysts). These initial sets of materials (catalysts, MOFs, COFs, ionic liquids) are then evaluated using in-house high-throughput synthesis and characterization setups. The synthesis conditions and corresponding material performances provide feedback to computational models to refine the material targets for further evaluation. The class of materials with better performance is then investigated using operando measurement and multiscale simulation techniques to understand underlying mechanisms and performance-limiting processes. This information is crucial to pushing the performance limits and discovering novel materials.
Discovery of Novel Processes
The evaluation of material performance (e.g., catalytic activity and selectivity, sorbent capacity and selectivity, and separators’ efficiency) at a process level is important to understand the integrability, robustness, and scalability of the process. Here, we first conduct full material and energy balance followed by techno-economic and life-cycle analysis to identify scale-limiting cost factors, which eventually result in necessary targets for materials performance and durability and process efficiency. We also perform a sustainability analysis to evaluate greenhouse gas (GHG) emissions of the process, which is a very important analysis to assess the overall feasibility of the process. The materials’ performance and durability targets provide feedback to the material discovery cycle, whereas the process efficiency targets can be achieved from novel process intensification schemes (e.g., integrating CO2 capture and conversion, CH4 capture and conversion, biochar oxidation with water electrolysis, direct air capture with cement production, etc.). These process intensification schemes will result in novel processes for energy and environment applications.
Summary of Active Research Projects
A summary of seven active and funded projects is listed below. These projects utilize our convergent research scheme in Fig. 1
1) Integrated CO2 Capture and Conversion to Ethylene
​​The proposed research plans to integrate electrochemical modeling & simulation, with experiments to address some of the outstanding challenges in the integration of carbon capture with the photo/electrochemical cells for CO2 conversion, which will substantially advance the development of integrated artificial photosynthesis (AP) systems. The broad objective of this research is to develop efficient catalysts and a prototype of a photo/electrochemical cell integrated with a carbon capture membrane for direct capture of CO2 from flue gas and its conversion to C2H4.
​
Aligned with: DOE Carbon Negative Shot, NAE GC – Develop Carbon Sequestration Methods
Partners: Braskem America, Prof. Jindal Shah (OSU), Prof. Anh Ngo (ANL), Prof. Joseph Gauthier (TTU)
Key Publications:
-
Prajapati et al., “Migration-assisted, moisture gradient process for ultrafast, continuous CO2 capture from dilute sources at ambient conditions,” Energy & Environmental Science, 15, 680-692, 2022,
-
Prajapati et al., “CO2-Free, High-Purity Ethylene from Electroreduction of CO2 on a 3D Cu Mesh with 4% Solar-to-Ethylene Efficiency,” Energy & Environmental Science, 2022 (under review)
2) Electrochemical Conversion of N2 to Nitrates and Ammonia at Near Ambient Conditions
​​Carbon neutral energy storage systems cannot be realized until technical barriers related to energy transportation, distribution, and storage are overcome. The concept of an NH3 economy has recently gained prominence for several reasons. First, NH3 is a cost-effective hydrogen carrier and carbon-free fuel is in a liquid state at near ambient conditions, and it enables a complete cycle of synthesis and consumption free of GHG emissions. Second, the infrastructure and supply chain for NH3 as a transport fuel already exists. Third, direct oxidation of NH3 to N2 via a fuel cell can be realized for electricity generation as a power source. Here we propose to meet this challenge by conducting fundamental research to drive materials discovery towards the identification of catalysts that will enable a circular nitrogen economy via interconversion of N2 and NH3, a critical feedstock chemical and viable carbon-free energy (density ~6.25 kWh kg-1) and hydrogen carrier (17.7 wt%, 40% higher than methanol).
​
Aligned with: DOE Long Duration Shot, NAE GC – Manage the Nitrogen Cycle
Partners: General Ammonia Co, Prof. Joseph Gauthier (TTU)
Key Publications:
-
Kani et al., “Solar-Driven Electrochemical Synthesis of Ammonia with >10% Solar-to-Fuel Efficiency at Ambient Conditions,” Energy & Environmental Science, 14, 6349-6359, 2021,
-
Kani et al., “Competing Effects of pH, Cation-Identity, H2O Saturation, and N2 Concentration on the Activity and Selectivity of Electrochemical Reduction of N2 to NH3 on Electrodeposited Cu at Ambient Conditions,” ACS Catalysis, 10 (24), 14592 – 14603, 2020.
3) Electrochemical Conversion of CH4 to Methanol and Formic Acid at Near Ambient Conditions
Our objective here are -
-
To address the critical issue of reigning in methane discharge into the atmosphere to combat climate change;
-
To make Oklahoma (OK) a leader in providing technology and application that is adaptable globally; and
-
Develop a plan for methane sequestration in collaboration with academia, industry, and the government to reach a targeted reduction goal for OK.
The proposed research activities include -
-
Map out all the plumes and quantities of methane discharges in the State of OK, which include oil production, oil refining, wastewater treatment facilities, animal farms, and landfills;
-
Advance methane capture and methane to methanol technology through RD&D efforts with an emphasis on OK;
-
Develop a plan for distributed application of the technology to reduce methane plume by 1 billion SCF annually on OK.
​
Aligned with: DOE Long Duration Shot, NAE GC – Develop Carbon Sequestration Methods
Partners: Prof. Jason D. Goodpaster (UMN), Prof. Joseph Gauthier (TTU), Prof. Jindal Shah (OSU)
Key Publications:
-
Prajapati et al., “Fundamental Insights into Electrochemical Activation of Methane towards Methanol over Transition Metal Oxides,” Proceedings of the National Academy of Sciences, 118 (8), e2023233118, 2021.
-
Prajapati et al., “Chloride-Promoted, High-Rate Ambient Electrooxidation of Methane to Methanol on a Patterned Cu-Ti Bimetallic Oxides,” ACS Catalysis (under review)
4) Biochar-Assisted Water Electrolysis for Production of Green Hydrogen
​​The proposed technology is a biochar-assisted water electrolysis (BAWE) process where biochar and H2O are oxidized at the anode to produce oxygenated carbon products, and the protons are migrated to the cathode to produce H2. The equilibrium potential for the oxidation of biochar is ~0.21 V which is six times lower than the water oxidation potential of ~1.23 V, thus offering significant savings in electrical power consumption and associated carbon emissions. To develop an efficient BAWE with the required performance, the PIs will rely on their extensive and demonstrated experience on biochar synthesis and combine it with patent-pending BAWE technology to actively and selectively produce H2 at current density > 100 mA/cm2 and cell potentials < 1 V. The innovative features of our technology includes (1) Pure H2 is produced without any side products at the cathode and oxygenated hydrocarbons (Oxy-HCs) are produced at the anode , (2) Theoretical H2 production could be six times that of traditional electrolysis of water for the same input of electrical energy, (3) “Green” hydrogen can be produced on-demand from renewable biomass, and (4) The process is mild temperature, ambient pressure, scalable and modularizable for various sizes at and various geographical locations around the world.
​
Aligned with: DOE Hydrogen Shot
Partners: Orochem Technologies Inc.
Key Publications:
-
Kani et al., “Solar-driven Biochar-Assisted Water Electrolysis for Green Hydrogen Production at Ambient Conditions with >30% STH Efficiency,” (in preparation)
5) Decarbonization of Cement Manufacturing
Decarbonization of the cement industry has been a longstanding environmental challenge because the bulk of the industry’s carbon dioxide emissions results from the chemical reaction that takes place during limestone calcining, the first step in traditional cement production. Produced by current practices, emissions associated with cement production alone would account for as much as 40% of the world’s carbon budget in 2050, a figure based on emissions allowable for a 50% chance of limiting warming to 1.5°C in 2050. To address this challenge, a novel calcination-free LoTeCH (Low-Temperature Calcium Hydroxide) process will be developed to produce calcium hydroxide that can replace limestone as the key cement precursor from distributed waste streams such as recycled concrete, coal ash, and metal-smelting slag. The resulting LoTeCH calcium hydroxide can be seamlessly integrated into the existing cement-making infrastructure to replace limestone calcination, reducing CO2 emissions by more than 50% with the added benefit of reduced limestone mining. In addition to LoTeCH technology, the team is also developing a carbon-negative replacement for portland cement, a component of concrete. This project will utilize direct air capture and convert industrial mineral wastes into a recyclable cementitious replacement for portland cement with superior durability. The process will store captured CO2 permanently as a strong, multi-purpose building material resulting in a net reduction in this greenhouse gas.
​
Aligned with: NAE GC – Develop Carbon Sequestration Methods
Partners: Prof. Bu Wang (U. Wisc. Madison [UWM]) and Prof. Robert Anex (UWM)
Key Publications:
-
Podupu et al., “A Comprehensive Model to Predict Leaching of Calcium ions from Concrete and Mineral Wastes,” (in preparation)
6) Efficient Computational Methods to Study Crystal Growth and Nucleation
Small molecules constitute almost 90% of today’s drug market, and a majority of these exist in solid crystalline form. After the discovery phase, it can take up to 5 years and a few million dollars to develop a robust manufacturing process for stable solid drugs. This is due to a lack of understanding of how small molecules self-assemble into a stable crystal form. In this project, we will integrate computations, experiments, and educational activities to advance the synthesis science of crystalline materials of small molecule active ingredients central to pharmaceuticals and agrochemicals. The objective is to identify molecular properties and mechanistic processes that govern solution crystallization and to develop strategies to control the self-assembly of molecules, leading to the formation of crystalline materials of desired features. Towards this goal, we will apply our emerging computational methods to study self-assembly mechanisms during cooling or anti-solvent crystallization of small organic molecules of increasing interaction complexities. It is anticipated that the fundamental knowledge garnered from this research will transform myriad applications, ranging from the synthesis of crystalline materials to the prevention of crystal growth.
​
Aligned with: DOE Carbon Negative Shot, NAE GC – Engineer better medicines
Partners: Prof. Gaurav Giri (UVA), Prof. Santanu Chaudhuri (ANL), Prof. Sapna Sarupria (UMN)
Key Publications:
-
Dighe et al., “Solvent Fluctuations in the Solvation-Shell Determines the Activation Barrier for Crystal Growth Rates,” Proceedings of the National Academy of Sciences, 116 (48), 23954 – 23959, 2019,
-
Dighe et al., “Autocatalysis and Oriented Attachment Direct the Synthesis of Metal-Organic Framework,” JACS Au, 2 (2), 453-462, 2022,
-
Dighe et al., “Selective Desolvation in Two-Step Nucleation Mechanism Steers Crystal Structure Formation,” Nanoscale, 14, 1723-1732, 2022.
7) Continuous-flow Microfluidic Platform for Screening of Crystalline Materials and High-Throughput Microtiter Plates for Screening Extreme Conditions
We have developed a novel microfluidic device that will help pharmaceutical companies more effectively screen and test formation of active pharmaceutical ingredient (API), and thereby reduce the process R&D efforts to get new drugs to market sooner. The industry currently uses microtiter plates (96-, 384-, and 1582-well plates) and droplet-based microfluidic devices to screen different crystalline forms of API. However, these devices suffer from depletion of supersaturation (driver for crystallization) due to nucleation and growth of API crystals in wells and droplets and hence biasing the screening results. This poses a huge risk when companies move to scale-up and technology transfer to manufacture APIs. An innovative technique is developed that uses cyclone mixer design in microfluidic chips to screen API at controlled supersaturations, which can be readily implemented on existing microtiter plates and thus providing a robust and indispensable tool for pharma companies.
​
Aligned with: DOE Carbon Negative Shot, NAE GC – Engineer better medicines
Partners: AbbVie Inc., BMS, Takeda, Biogen, Zoetis
Key Publications:
-
Coliaie et al., “In-Line Measurement of Liquid-Liquid Phase Separation Boundaries using a Turbidity-Sensor-Integrated Continuous-Flow Microfluidic Device,” Lab on a Chip (themed collection: Miniaturised Sensors and Diagnostics), 22, 2299-2306, 2022,
-
Coliaie et al., “On-the-Spot Quenching for Effective Implementation of Cooling Crystallization in Continuous-Flow Microfluidic Device,” Reaction Chemistry & Engineering, 7, 1179-1190, 2022,
-
Coliaie et al., “Machine-Learning-Driven, Sensor-Integrated Microfluidic Device for Monitoring and Control of Supersaturation for Automated Screening of Crystalline Materials,” ACS Sensors, 7 (3), 797-805, 2022.
Summary of Commercialization Efforts through PI’s Startup Company – eN-RAMPS
1) Deployment of Pilot Plant for CO2 Capture and Conversion to Ethylene
The planned efforts will be validated by developing a modular testbed that integrates a CO2 capture and conversion system, while utilizing simulated flue gas mimicking the actual flue gas coming from a commercial boiler (~1.7MW) emitting CO2 at 8.5 kmol/hr. The simulated flue gas will be utilized in the CO2 capture and conversion system for performance metrics evaluation for green ethylene production in an environment that will allow repeatable measurement and refinement of all aspects of the system at a pilot scale at the eN-RAMPS facilities specializing in sustainable manufacturing and prototyping, and having an established relationship with UIC. This goal will be pursued using an integrated approach that combines materials and process optimization, modular CO2 capture experiments using electrodialysis stacks, modular CO2 conversion experiments using electrolysis stacks, design and assembly of modular, fully-automated and scalable CO2 capture and conversion systems, integration of the CO2 capture and conversion system with simulated flue gas, and, finally, techno-economic analysis of the entire process.
​
Aligned with: DOE Carbon Negative Shot, NAE GC – Develop Carbon Sequestration Methods
Partners: Braskem America
2) Modular CO2 Capture Unit for Residential/Commercial Scale
The objective of this project is to develop a compact, modular CO2 capture unit for residential/commercial applications. This unit integrates an air contactor with an electrodialysis unit to capture CO2 from the recirculated air in the building to keep the fresh air intake to a minimum level.
​
Aligned with: DOE Carbon Negative Shot, NAE GC – Develop Carbon Sequestration Methods
Partners: RZero
3) eN-XTAL: An Automated Platform for Screening of Crystalline Materials
Our project with ETC resulted in an advanced microfluidic device, which is currently at TRL 7. The research planned for the next 24-month project with ETC will help in the automation of the device, which will bring our technology to TRL 8+ level (closer to commercialization). The objective here is to develop and deploy a standalone fully-automated microfluidic system to measure crystal growth rate and nucleation rate, screen polymorph, and assess solid-liquid and liquid-liquid equilibrium, which will be seamlessly coupled with a graphical user interface to characterize and optimize the crystallization process quickly and reliably. We will develop and implement electrochemical sensors to measure supersaturation, Raman sensor for polymorph identification, an optical sensor for turbidity (induction time and hence nucleation rate), and growth rate measurements in our continuous-flow microfluidic device. We will interface the sensors and fluid delivery systems with snap-on microfluidics, which will sit on a motorized xyz translation stage system to automate the screening process.
​
Aligned with: NAE GC – Engineer better medicines
Partners: AbbVie Inc., BMS, Takeda, Biogen, Zoetis